Research
Current Research at the Rando Lab
Stem cells exist in most organs in the body and are responsible for tissue homeostasis and repair. These rare cells hold the promise of novel therapeutics for degenerative diseases, for which there are currently no cures or even effective treatments. Many questions remain unanswered about how stem cell functions are regulated and impacted by changes in the local and systemic environment during aging or in disease conditions. Using skeletal muscle and the resident muscle stem cells as a model system, our laboratory seeks to understand how stem cells function, how they change with age, and how to identify means to restore youthful function to aged stem cells. We take a multi-omics approach to identify critical factors and pathways that regulate fundamental features and processes of muscle stem cells, including the maintenance of quiescence and self-renewal, and we use various mouse models and bioengineering approaches to explore stem cell function and regenerative potential in vivo. We use gene editing approaches (CRISPR) bot for screening and for specific genetic modifications to study stem cell function. Below we list representative projects that are ongoing in the laboratory and that reflect our fundamental scientific questions, underlying hypotheses, and translational goals.
Stem cells in muscle regeneration
A long-standing interest of the laboratory has been in the complex biology of tissue repair. Skeletal muscle regeneration involves the coordinated responses of muscle stem cells, fibroadipogenic progenitors, vascular endothelial cells, and cells of the immune system, followed by reinnervation of regenerating muscle fibers by axons of motor neurons. We have generated many genetic models to trace individual cell populations or to delete or induce expression of genes in a cell type-specific manner. Using these models, we have explored signaling pathways, such as the Notch and Wnt pathways, that are critical in coordinating muscle stem cell activation, proliferation, and differentiation. We also study the essential role of fibroadipogenic progenitors in the regenerative response and in normal tissue homeostasis. We will continue to explore the complex interaction between these cell types in the muscle stem cell niche and how to harness these interactions to enhance regenerative responses.
Liu L, Rodriguez-Mateo C, Huang P, Huang A, Lieu A, Mao M, Chung M, Yang S, Yu K, Charville GW, Gan Q, Rando TA (2021) Hairless regulates heterochromatin maintenance and muscle stem cell function as a histone demethylase antagonist. Proc Natl Acad Sci, USA, 118: e2025281118.
Wosczyna MN, Perez Carbajal EE, Wagner MW, Paredes S, Konishi CT, Liu L, Wang TT, Walsh RA, Gan Q, Morrissey CS, Rando TA (2021) Targeting microRNA-mediated gene repression limits the adipogenic conversion of skeletal muscle mesenchymal stromal cells. Cell Stem Cell, 28, 1323-1334.
de Morree A, Klein JDD, Gan Q, Farup J, Urtasun A, Kanugovi A, Bilen B, van Velthoven CTJ, Quarta M, Rando TA (2019) Alternative polyadenylation of Pax3 controls muscle stem cell fate and muscle function. Science, 366: 734-738
Wosczyna MN, Konishi CT, Perez Carbajal EE, Wang TT, Walsh RA, Gan Q, Wagner MW, Rando TA (2019) Mesenchymal stromal cells are required for regeneration and homeostatic maintenance of skeletal muscle. Cell Reports, 27: 2029-2035.
Wosczyna MN, Rando TA (2018) A muscle stem cell support group: Coordinated cellular responses in muscle regeneration. Devel Cell, 46: 135-143.
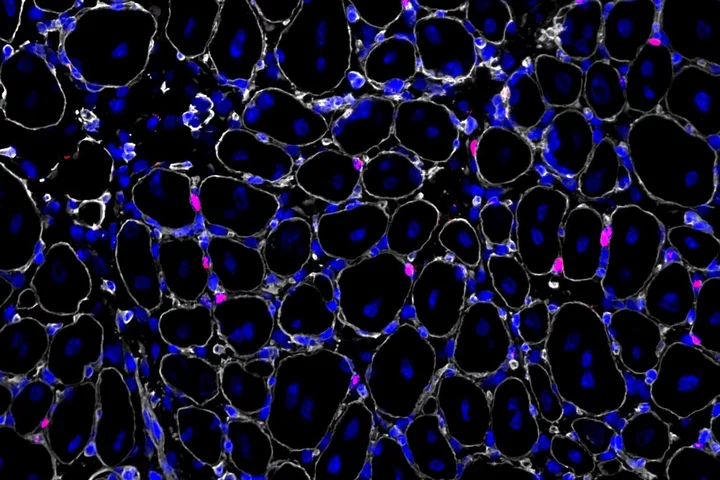
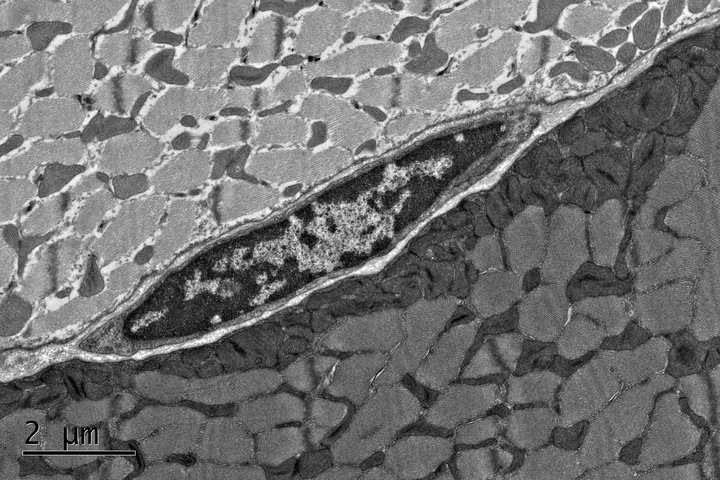
Stem cell quiescence
Muscle stem cells persist in a quiescent state in muscle tissue in the absence of any injury, and we have studied many aspects of the biology of quiescence. One of our main contributions to this field has been the demonstration that the quiescent state of stem cells is actively maintained. This has become a paradigm in the stem cell field, supplanting the older notion that quiescence is a state of dormancy. We have shown that quiescence is actively maintained transcriptionally and post-transcriptionally. Disruption of critical factors or pathways that maintain quiescence leads to their spontaneous exit from quiescence and entry into the cell cycle, in many cases resulting to a gradual depletion of stem cells. We have also explored the dynamics of the quiescent state, demonstrating that muscle stem cells can adopt stable states of deeper or shallower quiescence in response to systemic cues. Our current studies are focused on the regulation of signaling pathways that promote the return to quiescence of muscle stem cells that have entered the cell cycle, as well as methods to interrogate quiescence in vitro.
de Morrée A, Rando TA (2023) Regulation of adult stem cell quiescence and its functions in the maintenance of tissue integrity. Nature Rev Mol Cell Biol, 24: 334-354.
Benjamin DI, Both PA, Benjamin JS, Nutter CW, Tan JH, Kang J, Machado LA, Klein JDD, de Morree A, Kim S, Liu L, Dulay H, Feraboli L, Louie SM, Nomura DK, Rando TA (2022) Fasting induces a highly resilient deep quiescent state in muscle stem cells via ketone body signaling. Cell Metabol, 34: 902-918.
Salvi JS, Kang J, Kim S, Colville AJ, de Morree A, Bileskov T, Larsen MC, Kanugovi A, van Velthoven CTJ, Cimprich KA, Rando TA (2022) ATR controls stem cell quiescence via the Cyclin F-SCF complex. Proc Natl Acad Sci, USA, 119: e2115638119.
Arjona M, Goshayeshi A, Rodriguez-Mateo C, Brett JO, Both P, Ishak H, Rando TA (2022) Tubastatin A maintains adult skeletal muscle stem cells in a quiescent state ex vivo and improves their engraftment ability in vivo. Stem Cell Rep, 17: 82-95.
van Velthoven, Rando TA (2019) Stem cell quiescence: Dynamism, restraint, and cellular idling. Cell Stem Cell, 24: 213-225.
van Velthoven CTJ, de Morree A, Egner IM, Brett JO, Rando TA (2017) Transcriptional profiling of quiescent muscle stem cells in vivo. Cell Reports, 21: 1994-2004.
de Morree A, van Velthoven CTJ, Gan Q, Salvi JS, Klein JDD, Quarta M, Biressi S, Rando TA (2017) Staufen1 inhibits MyoD translation to actively maintain muscle stem cell quiescence. Proc Natl Acad Sci, USA, 114: E8996-E9005.
Cellular aging and epigenetic rejuvenation
One of the main interests of our laboratory has been the molecular drivers of cell and tissue aging and how different interventions (genetic, pharmacologic, lifestyle) may impact the aging process or potentially reverse aging phenotypes. In the early 2000’s, we pioneered the use of heterochronic parabiosis in the study of cell and tissue aging and rejuvenation. These studies highlighted the role of the local and systemic milieu in determining changes in the functionality of stem cells with age. This led to a tremendous expansion of research in this area, with studies focusing on blood-borne factors that either promote or reverse cellular aging and on the epigenetic features associated with these changes. The notion of epigenetic rejuvenation became widely accepted and has been supported by studies showing reprogramming of cellular age by a wide variety of interventions. We are currently exploring how lifestyle interventions such as diet and exercise may promote youthfulness by similarly reprogramming cellular biological age and how metabolic changes may influence the epigenetic state of cellular age. In parallel, we are using variations of cellular reprogramming with Yamanaka factors, or molecular mimics, to explore the potential of epigenetic rejuvenation via partial reprogramming.
Colville A, Liu J-Y, Rodriguez-Mateo C, Thomas S, Ishak HD, Zhou R, Klein JDD, Morgens DW, Goshayeshi A, Salvi JS, Yao D, Spees K, Dixon SJ, Rhee JW, Lai C, Wu JC, Bassik MC, Rando TA (2023) Death-seq identifies regulators of cell death and senolytic therapies. Cell Metabol, 35: 1814-1829.
Liu L, Kim S, Buckley MT, Reyes JM, Kang J, Tian L, Wang M, Lieu A, Mao M, Mateo-Rodriguez C, Ishak HD, Jeong M, Wu JC, Goodell MA, Brunet A, Rando TA (2023) Exercise reprograms the inflammatory landscape of multiple stem cell compartments during mammalian aging. Cell Stem Cell, 30: 689-705
Benjamin DI, Brett JO, Both P, Benjamin JS, Ishak HL, Kang J, Kim S, de Morree A, Arjona M, Nutter CW, Tan JH, Dulay H, Louie SM, Nomura DK, Rando TA (2023) Multiomics reveals glutathione metabolism as a driver of bimodality during stem cell aging. Cell Metabol, 35: 472-486
Brunet A, Goodell MA, Rando TA (2023) Ageing and rejuvenation of tissue stem cells and their niches. Nature Rev Mol Cell Biol, 24: 45-62.
Rando TA, Wyss-Coray T (2021) Asynchronous, contagious, and digital aging. Nature Aging, 1: 29-35.
Brett JO, Arjona M, Ikeda M, Quarta M, de Morrée A, Egner IM, Perandini LA, Ishak HD, Goshayeshi A, Benjamin DI, Both P, Rodriguez-Mateo C, Betley MJ, Wyss-Coray T, Rando TA (2020) Exercise rejuvenates quiescent skeletal muscle stem cells in old mice through restoration of Cyclin D1. Nature Metabol, 2: 307-317.
Liu L, Charville GW, Cheung TH, Yoo B, Santos PJ, Schroeder M, Rando TA (2018) Impaired Notch signaling leads to a decrease in p53 activity and mitotic catastrophe in aged muscle stem cells. Cell Stem Cell, 23: 544–556.
Brunet A, Rando TA (2017) Interaction between epigenetics and metabolism in aging stem cells. Curr Opin Cell Biol, 45: 1-7.
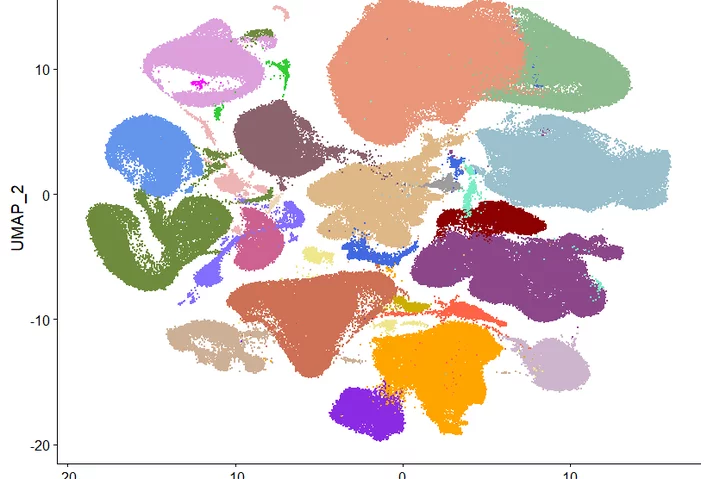

Muscular dystrophies and fibrosis
Muscular dystrophies are genetic, degenerative diseases of skeletal muscle, and our laboratory has had a long-standing interest in understanding the pathogenesis of these disorders and exploring experimental therapeutics. We have developed mouse models for non-invasively monitoring disease activity in living animals. Studies from our laboratory have identified signaling networks and cell types that can lead to excessive tissue fibrosis in animal models of muscular dystrophy. These studies are relevant not only to the fibrosis that accompanies degenerative diseases, but also to that seen during aging and in response to severe injury. We are expanding on our previous studies exploring the role of Wnt signaling in driving fibrosis with age, and we are examining the role of complement proteins in the pathogenesis of fibrosis.
Florio F, Vencato S, Papa FT, Libergoli M, Kheir E, Ghzaiel I, Rando TA, Torrente Y, Biressi S (2023) Combinatorial activation of the WNT-dependent fibrogenic program by distinct complement subunits in dystrophic muscle. EMBO Mol Med, 15: e17405.
Biressi S, Filareto A, Rando TA (2020) Stem cell therapy for muscular dystrophy. J Clin Invest, 130: 5652-5664.
Filareto A, Nguyen KM, Gan Q, Aldanondo G, Machado L, Chamberlain JS, Rando TA (2018) Monitoring disease activity non-invasively in the mdx model of Duchenne muscular dystrophy. Proc Natl Acad Sci, USA, 115: 7741-7746.
Mueller AA, van Velthoven CT, Fukumoto K, Cheung TH, Rando TA (2016) Intronic polyadenylation of PDGFRα in resident stem cells attenuates muscle fibrosis. Nature, 540: 276-279.
Tissue engineering
We have pursued projects in tissue engineering, focusing primarily on stem cell transplantation in combination with materials science, to promote tissue repair in vivo. Using a model of muscle trauma, namely volumetric muscle loss (VML), we have demonstrated the importance of bioscaffold properties and cellular composition in promoting effective tissue repair in both acute and chronic VML lesions. We have tested characteristics such as stiffness and porosity of bioscaffolds to optimize the environment for stem cell function, and we have incorporated physical activity in a “regenerative rehabilitation” approach to enhancing functional recovery. Our current studies are focusing on using engineered nanocapsules to control the timed and sustained delivery of factors to promote all aspects of muscle wound healing, including myogenesis, angiogenesis, and innervation.
Eugenis I, Wu D, Hu C, Chiang G, Huang NF, Rando TA (2022) Scalable macroporous hydrogels enhance stem cell treatment of volumetric muscle loss. Biomaterials, 290: 121818.
Eugenis I, Wu D, Rando TA (2021) Cells, scaffolds, and bioactive factors: Engineering strategies for improving regeneration following volumetric muscle loss. Biomaterials, 278: 121173
Quarta M, Lear MJC, Blonigan J, Paine P, Chacon R, Rando TA (2018) Biomechanics show stem cell necessity for effective treatment of volumetric muscle loss using bioengineered constructs. NPJ Regen Med, 3: 18.
Rando TA, Ambrosio F (2018) Regenerative rehabilitation: Applied biophysics meets stem cell therapeutics. Cell Stem Cell, 22: 306-309.
Quarta M, Cromie M, Chacon R, Blonigan J, Garcia V, Akimenko I, Hamer M, Paine P, Stok M, Shrager JB, Rando TA (2017) Bioengineered constructs combined with exercise enhance stem cell-mediated treatment of volumetric muscle loss. Nature Commun, 8: 15613.
Quarta M, Brett JO, DiMarco R, de Morree A, Boutet SC, Chacon R, Gibbons M, Garcia VA, Su J, Heilshorn S, Rando TA (2016) An artificial niche preserves the quiescence of muscle stem cells and enhances their therapeutic potential. Nature Biotechnol, 34: 752-759.
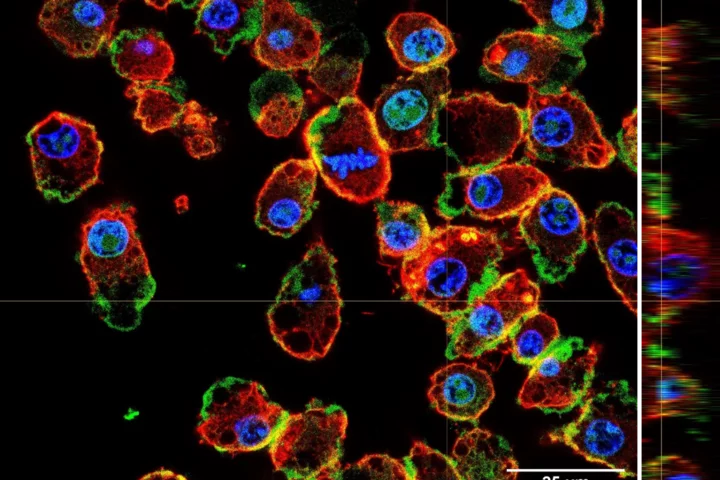